Vision & the Eye
How is vision created?
Electromagnetic radiation takes many forms, ranging from low-energy radio waves to high-energy gamma rays. Only the middle wavelengths have enough energy for vision, but not so much that they can damage tissue. This relatively narrow band of wavelengths that so many unrelated kinds of organisms use to gather information about their environments is the portion of the electromagnetic spectrum that we call light.
Light receptors in animals
Almost all animals respond to light. Even some protozoa react quickly to changes in light intensity, often moving toward or away from brightly lit areas. These organisms have a specialized region containing pigment that undergoes chemical changes when exposed to light.
The light-sensitive pigment common in many animals is a protein attached to a portion of a carotenoid molecule. There are over 600 known carotenoids which are yellow or orange light-sensitive pigments found in chloroplasts and chromoplasts of plants, some bacteria and fungi. Animals must obtain carotenoids in their diet as a vitamin (vitamin A).
The spectrum of vision
The light receptors of many invertebrates do not function as eyes in the usual sense of the word. Some of these relatively simple receptors do nothing more than indicate the general light intensity.
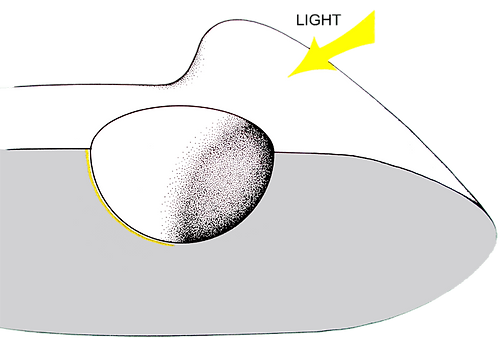
planarian eye cup
The planarian eye cup provides directional information that the organism can use to maneuver in the environment; it does not form images. The direction of the light source is indicated by the location of the shadow cast by the cup’s opaque edge onto receptors within the organ.
Two considerably more complex strategies enable most animals to see images of the world about them. These are the compound eye and the camera eye.
Compound eyes
The compound eye uses what is basically an array of tiny eye cups, each modified into a tube, called an ommatidium, which points out at the world in a slightly different direction.
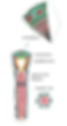
Ommatidia from the compound eye of a typical diurnal insect
Top: Section of compound eye. Bottom: Longitudinal and cross sections of one ommatidium. The lens and crystalline cone focus incoming light rays into the rhabdom, a translucent cylinder formed by the highly specialized microvilli in the rhabdomeres of the eight receptor cells. Photosensitive pigment is located in the microvilli, and the pigment cells surrounding the ommatidium contain a dark pigment that prevents passage of light from one ommatidium to another.
Light entering through the lens and crystalline cone of each ommatidium is focused onto an array of seven to nine elongated receptor cells. With so many layers for light to pass through, compound eyes are more efficient at absorbing photons than the camera eyes we shall examine next.

The compound eye of a horse fly
Each eye is composed of a huge number of ommatidia
In many species with compound eyes the individual rhabdomeres have pigment that absorb maximally (and therefore most sensitive to) light from particular parts of the spectrum. In honey bees, for instance, there are three pigments in the cells of each ommatidium: two cells have a pigment that absorbs green light most effectively, two cells absorb primarily blue light, three respond best to ultraviolet (UV), and the last two absorb either green (if the ommatidium is in the ventral half of the eye, where it will most often see vegetation) or UV (if in the dorsal half, where it will normally view the sky). The differing sensitivities of these three pigments, tuned respectively to green, blue, and UV light, from the basis of color vision in most insects.
The picture produced by an insect brain from the information it receives from the compound eye is probably a grainy mosaic of the world, with far less precise delineation of objects in the visual field than we experience. Several advantages of compound eyes, however, compensate for this low spatial resolution. Besides being very efficient at absorbing light, they are very small and lightweight, which is important for the flying insect. In addition, most compound eyes permit arthropods to see details of movements that are far too rapid for our eyes.

Exemplified view through a compound eye
Camera eyes
There are two versions of the camera eye. By far the rarer of the two is the pinhole eye of organisms such as the chambered nautilus.
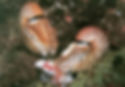
Cephalopods such as these juvenile nautili, seen feeding on fish, have pinhole eyes.
The pinhole eye is a covered eye cup with a tiny opening in its surface. Light from the world outside passes through the hole and is projected onto the array of receptors (the retina) at the back. There is no need for a lens, but a great disadvantage is that only little light can enter; if the opening were widened to admit more light, the image would become blurred.

How a pinhole eye works
Only the focused light reflected from an object in the environment is able to pass through the pinhole and projects a precise inverted image on the retina. The main disadvantage of this strategy is the need for relatively strong ambient light in order for an image to be projected. Animals with pinhole eyes cannot see very well under the glimmering moonlight.
The lens eye, on the other hand, can have a far larger opening for light, since the lens focuses all incoming light on the retina.

How a lens eye works
The lens eye admits much more light than the pinhole eye, but has great constraints on its focusing ability. Unlike a pinhole eye, a lens eye can focus on objects at only one distance at any one time. Mammals, birds, and some reptiles have muscles to change the shape (and thus the focus) of their lenses, while fish, amphibians, and other reptiles move the whole lens toward or away from the retina like a true camera. Moreover, the retina and lens must have just the right spatial relationship: if the retina is too close to the lens, the animal will have difficulty seeing nearby objects (that is, it will be farsighted), whereas if the retina is too far away, distant objects cannot be brought into focus (the animal is nearsighted). In addition, irregularities in the lens itself may give rise to astigmatism, in which images of objects at the same distance from the lens but in different parts of the visual field do not come into focus concurrently. An insuperable disadvantage of the camera eye for many animals is its bulk: a camera eye large enough to provide the same visual resolution as the compound eye of a honey bee would weigh more than the bee itself.
The human eye
The adult human eye is a globe-shaped lens eye with a diameter of about 2.5cm. It is encased in tough but elastic coat of connective tissue, the sclera. The anterior portion of the sclera, the cornea, is transparent and more strongly curved. The cornea functions as the first element in the light-focusing system of the eye. Just inside the sclera is a layer through which many blood vessels run, the choroid. The choroid is important both as the structure providing blood supply to the eye and as a light-absorbing layer. Between the choroid and the retina lies a pigment layer that, like the black inner surface of a camera, also helps prevent the internally reflected light from blurring the image. In nocturnal animals, by contrast, this layer is usually highly reflective. It increases sensitivity by sending unabsorbed light back through the receptor layer for another try. This mirror like layer accounts for the way that cat’s eyes seem to glow in the dark, for example.

Just behind the junction between the main part of the sclera and the cornea, the choroid becomes thicker and has smooth muscles embedded in it; this portion of the choroid is called the ciliary body. Anterior to the ciliary body, the choroid leaves the surface of the eye as a ring of pigmented tissue, the iris. The iris contains smooth muscle fibers arranged both circularly and radially. When the circular muscle fibers contract, the opening of the center of the iris (the pupil) is reduced. When the radial muscles contract, the pupil is dilated. The iris thus regulates the amount of light admitted to the eye in much the same way that the diaphragm of a camera controls the lens aperture.
The lens, which functions as the second element in the light-focusing system, is suspended just behind the pupil by a suspensory ligament made of zonules and attached to the ciliary body. The exact shape of the lens is controlled by the tension applied by an array of tiny muscles mounted in the ciliary body. The lens and its suspensory ligament divide the cavity of the eyeball in two. The cavity between the cornea and the lens is filled with a watery fluid, the aqueous humor. The segment behind the lens is filled with gelatinous material, the vitreous humor.
The retina, which contains the receptor cells, is a thin tissue covering the inner surface of the choroid. It is composed of several layers of cells: the receptors, sensory neurons, and interneurons. The receptors are of two types, rods and cones.

Rod and cone cells
Retina cells under scanning electron microscope
The rod cells are more abundant toward the periphery of the retina, and are extremely sensitive to light; they allow us to see in very dim conditions, but produce colorless, poorly defined images. The cone cells, which are specialized for color vision, require bright light to function. They are especially abundant in the central portion of the retina, areas known as the macula and fovea. Because of the ultra-high density of receptors in the fovea, we are able to see a narrow area in the center of the visual field in very fine detail.
The rods and cones synapse in the retina with short sensory neurons (bipolar cells), which themselves synapse with the retinal ganglion cells, whose axons bundled together as the optic nerve, run to the visual centers of the brain.
The interconnection of neurons in the retina enables the eye to modify extensively the information transmitted from roughly a hundred million receptor cells through one million or so axons of the optic nerve to the brain. Oddly enough, in most vertebrates these processing cells lie between the lens and the receptors, a location that requires a sizable hole in the retina for the optic nerve fibers to pass through. In squid, octopus, and snakes, the processing cells are behind the receptors, an arrangement that creates no blind spot in their vision.
The light sensitivity of rods and cones
Both rods and cones contain light-sensitive pigments. In rods the pigment which is built into membranes of the flattened vesicles in the outer segment, is called rhodopsin. It contains a protein (opsin) bounded to a prosthetic group called retinol, which is a derivative of vitamin A. When a molecule of rhodopsin is struck by a photon of light, the retinol is converted into a slightly different isomer.

The light driven change of retinol from one isomer to another
This conversion is entirely light driven; no enzyme is required. The isomerization of the retinol leads, in turn, to conformational changes in the protein and to hydrolysis of the bond between the retinol and the protein. The result is a change in the polarity of the plasma membrane of the rod cell, which alter transmitter release at synapses between the rod cell and the bipolar cells (or the horizontal cells). In the dark, retinol is reconverted to the original isomer and rebounded to opsin to form functional rhodopsin.
The mechanism of cone vision is much more complex. There are three classes of cones, each containing a different pigment. All three human pigments have retinol as their prosthetic group, and all three absorb light over a wide range of wavelengths. However, their protein components differ slightly, and as a result, the range of wavelengths for each pigment centers on a different part of the spectrum.

Absorption spectra of the three cone pigments
The red-absorbing pigment actually has its maximum sensitivity in the yellow region of the spectrum. The relative narrowness of the absorption spectrum of the blue-absorbing cone is a result of faint yellow pigments in the lens, which absorb ultraviolet light. Current theory suggests that in processing color vision the nervous system compares and contrasts the output of the cones in pairs, to obtain the fine color distinctions we consciously perceive.
We are so accustomed to the human version of color vision that we tend to assume that the colors we see are somehow “true,” and that other animals must see the world in the same way we do. Humans and other primates, however, are rather unusual among the mammals in processing well-developed color vision. Most mammalian species see the world in varying shades of gray. By contrast, most birds and many fish and reptiles appear to have color vision, and some even detect light in the UV range.